Why GPS Systems Might Miscalculate Your Athlete’s Mechanical Load
Musculoskeletal tissues; such as bone, muscle, tendon and cartridge, respond and adapt to their local mechanical environment in such a manner as to maintain homeostasis. Knowledge of how an individual tissue responds and adapts to stress is critical when designing athlete movement analysis systems. This maximises performance gains and minimises risk of athlete injury. Given an appropriate loading stimulus and sufficient rest and recovery, musculoskeletal tissue will adapt positively to load. However, if you miscalculate athlete mechanical load, have mismatched loads or lack of recovery – these can also result in injury. Lower limb musculoskeletal injuries are common in competitive sport. As all have a mechanical aetiology, either via acute mechanisms (e.g. single impact event) or via repetitive, chronic mechanisms (e.g. fatigue fracture or tendinopathy).
Before we go any further, it is prudent to know the difference between two terms which are commonly spoken about in research and applied settings; external and internal workload. External workload can be thought of as how much load is placed on the body and is often followed by descriptions of various wearable technologies such as global positioning systems (GPS). Conversely, internal workload therefore, describes the forces which are acting on the individual tissues in the body and how those forces are distributed.
Recent research by Edwards et al [2], concluded that the placement of the inertial accelerometer within the elasticated harness at the thoracic region of the spine, produced poor reliability and validity compared to the gold standard. Despite this, trunk mounted accelerometers are commonly used to quantify sports performance variables such as vertical ground reaction forces (vGRF).
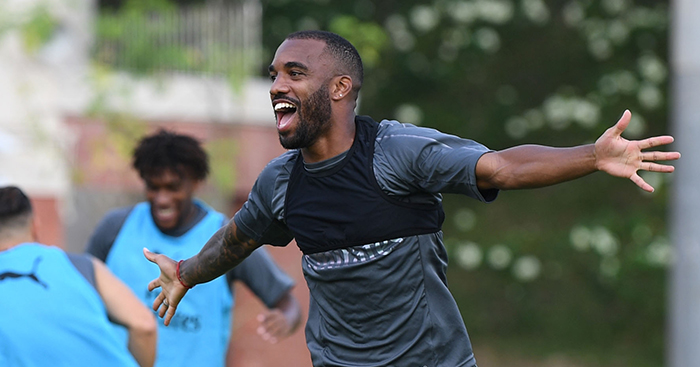
Photo courtesy of Planet Football
The terms ‘acute’ and ‘chronic’ have also been used to describe bouts of athlete training, where the ratio of acute: chronic workload has been shown to be predictive of performance gains [1]. In this context, athlete workload was determined using a torso-mounted global positioning system (GPS) (to measure speed and distance traveled) and a surrogate measure of player load using a tri-axial accelerometer (i.e. to measure step count). While informative, GPS data and accelerations of the torso may not be effective in monitoring loads of the lower limbs. This is because distance and speed traveled do not represent the mechanical load experienced by the musculoskeletal tissue. This is particularly relevant in small-space sport situations; or sports such as basketball, where players can experience high loads of stress by performing explosive jumping and landing activities, which are not captured by distance, speed, or torso athlete movement analysis systems.
Tri-axial accelerometers are often housed in player tracking devices in an attempt to negate some of the issues outlined above. However, recent research by Edwards et al [2], concluded that the placement of the inertial accelerometer within the elasticated harness at the thoracic region of the spine produced poor reliability and validity compared to the gold standard. Despite this, trunk mounted accelerometers are commonly used to quantify sports performance variables such as vertical ground reaction forces (vGRF). However, player tracking athlete accelerometry variables are often not sensitive enough to detect changes in athlete running speed. This is an obvious problem in intermittent team sports such as football, rugby, basketball, and tennis. Accelerometers placed on the extremities e.g. tibia allow for the measurement of tibial shock or peak impact accelerations which can function as a surrogate measure of the loads experienced by the underlying musculoskeletal tissue [3,4]. This simple, noninvasive method has been used across studies looking at impacts in landing, running, and walking tasks [4,5].
IMeasureU captures these impact events from two synchronized sensors at 500 Hz (frames/sec), thus providing accurate measurement of the impact loading placed on both limbs of the athlete. In order to adequately identify cumulative lower limb load, an appropriate metric is required which takes in to account the association between the musculoskeletal tissue and mechanical load (i.e. a mechanobiological approach). The daily load stimulus (DLS), is a metric of cumulative load which describes the relationship between mechanical load stimuli and skeletal tissue remodeling as a function of the stimulus [6]. The DLS is the product of the magnitude of the loads and the number of load cycles (or frequency) of these loads.
Importantly, the load magnitudes are weighted by an exponential component. This is to account for the greater influence the magnitude of the strain has on skeletal tissue relative to the impact of the frequency/number of load cycles [6]. The combination of all the varying loads and their cycles provides a total load stimulus or cumulative load. This mechanobiology concept has been used to predict bone remodeling and can also be applied to other skeletal tissue (e.g. cartilage and tendon) to prevent sports injuries. By integrating IMeasureU sensor technology with this mechanobiological perspective of tissue adaptation, the tools can accurately measure and monitor the mechanical workload of an individual basketball or football athlete.
From a return-to-play point of view, it may be useful to know how far an athlete has run or how fast they have run, however, coaches need to accurately measure not only the magnitude of load but the cycles of load that the athlete is experiencing. It is essential in this scenario for the athlete to wear one step sensor per leg to enable a coach to determine if there are any asymmetries which may indicate that the athlete is not ready to progress to the next stage of rehabilitation. Through the data captured by IMeasureU, coaches are able to monitor the intensity of every step the athlete takes and through our knowledge of bone load, we know that the intensity is much more important than the cycles of load. This load can then be tracked over time to enable a safe and effective return to play for the athlete.
When measuring inertial mechanical load, whether that be to maximize performance gains or minimize injury risk, it is essential to know the benefits and limitations of the technology which is available. Although accelerometers housed within player tracking devices may be convenient, caution is advised when using trunk mounted accelerometer data to quantify and monitor athlete workloads, as they might not provide the whole picture.
Read the follow-up article Why GPS systems may be miscalculating your athlete’s mechanical load – Part 2
References
- Murray N.B., Gabbett T. J., Townshend A.D., Hulin B.T., & McLellan C. . (2016). Individual and combined effects of acute and chronic running loads on injury risk in elite Australian footballers. Scandinavian Journal of Medicine and Science in Sports.
- Edwards, S., White, S., Humphreys, S., Robergs, R., & O’Dwyer, N. (2018). Caution using data from triaxial accelerometers housed in player tracking units during running. Journal of Sports Sciences.
- Zhang S., Derrick T.R., Evans W., et al. (2008). Shock and impact reduction in moderate and strenuous landing activities. International Society of Biomechanics in Sports.
- Hamill J., Derrick T.R., and Holt K.G. (1995). Shock Attenuation and Stride Frequency during Running. Human Movement Science.
- Lafortune, M.A. Three-dimensional acceleration of the tibia during walking and running. Journal of Biomechanics.
- Beaupre G.S., Orr, T.E., & Carter, D.R. (1990). An approach for time-dependent bone modeling and remodeling-application: a preliminary remodeling simulation. Journal of Orthopaedic Research.
I really like this article