If you’re trying to track mechanical load, GPS systems won’t give you the whole picture
This article is a follow up to Why GPS systems may be miscalculating your athlete’s mechanical load – Part 1
Training aims to induce physical adaptations for enhanced performance whilst reducing the risk of injury. However to increase endurance, speed, strength and/or power, a sufficient training stimulus is needed. But an excessive training load can put an individual at risk of injury. To prescribe adequate training loads for the desired adaptations, it is critical to have a thorough understanding of the relationship between the loads experienced by the body and the physical adaptations. GPS systems do not analyze this. Therefore, the physiological and biomechanical load-adaptation pathways could be evaluated separately [1]
“Historically, biomechanical loads have been poorly quantified in the field and are thus not well understood. Companies like IMeasureU, now part of Vicon, are bridging the gap between the lab and the field and enabling investigations that accurately quantify biomechanical loads”
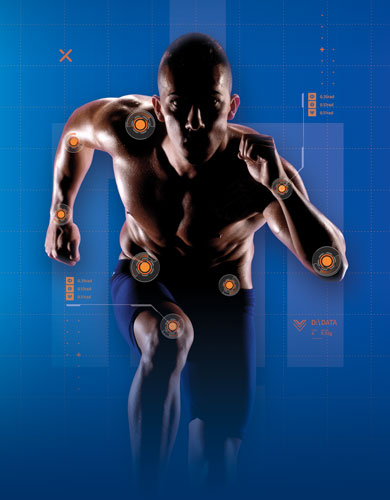
GPS Limitations
Physiological loads can be defined as the biochemical stresses experienced by the cardiovascular system. Enough stimuli may lead to desired adaptations such as increased metabolic and/or cardiac efficiency, while an over or under load could cause energy depletion or cardiac atrophy. Examples of commonly used measures to assess the internal physiological loads include oxygen uptake (VO2), heart rate, blood lactate and/or self-reported ratings of perceived exertion (RPE). The external physiological loads, on the other hand, are commonly quantified by GPS-derived metrics such as the total distance covered or time at certain speed or acceleration thresholds. Although these variables may be used to quantify and evaluate the aerobic and metabolic demands of training, they do not describe the loads experienced by the musculoskeletal system [2].
Advances in Technology
Biomechanical loads can be defined as the forces and stresses (i.e. normalized, distributed force) acting on the various hard and soft tissues (e.g. muscles, tendons, bones, cartilage) of the body. The repetitive mechanical loading of subsequent training sessions causes tissue-damage, which is necessary for positive adaptations such as stiffer tendons or muscle hypertrophy. However, an accumulation of damage over time can progressively weaken the tissues and ultimately lead to failure of a particular structure (i.e. overuse injuries) [3]. In contrast to the physiological loads, the biomechanical demands of training have been historically difficult to measure in the field. This limits detailed descriptions of the biomechanical load-adaptation pathways. However, recent advances in technology such as small, synchronized inertial sensors are enabling scientists, practitioners, and coaches to accurately measure, and therefore manage, biomechanical load in the field. Scientists, once previously constrained to the lab, can now work alongside practitioners, coaches and athletes to measure, analyze, and action field-based insights. Performance, return to play programming and injury risk reduction can then be improved with this data. The tech is here but up until now, what’s been missing is aligning sensors, software, and science. We now have a way to directly link sensor data to known underlying biomechanical models, and then present it in a simple, actionable way to practitioners, coaches, and athletes.
What Does the Research Say?
Field-based Internal Loading Assessment
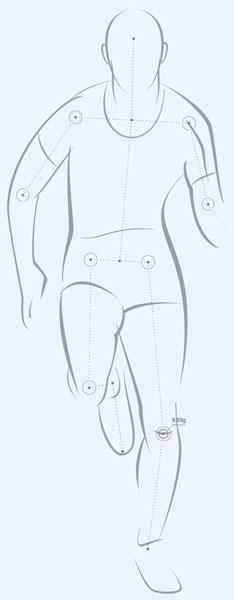
Conclusion
Interested in how IMU Step can help you quantify mechanical load and remove the guesswork from your own RTP protocols? Click here to learn more.
-
Vanrenterghem J., Nedergaard N.J., Robinson M.A., Drust B. (2017) Training Load Monitoring in Team Sports: A Novel Framework Separating Physiological and Biomechanical Load-Adaptation Pathways. Sports Medicine.
-
IMeasureU. (2018) Why GPS systems may be miscalculating your athletes mechanical load
-
Edwards B. (2018) Modeling Overuse Injuries in Sport as a Mechanical Fatigue Phenomenon. Exercise and Sport Sciences Reviews.
-
Nedergaard N.J., Robinson M.A., Eusterwiemann E., Drust B., Lisboa P.J., Vanrenterghem J. (2017) The Relationship Between Whole-Body External Loading and Body-Worn Accelerometry During Team-Sport Movements. International Journal of Sports Physiology & Performance.
-
Raper D.P., Witchalls J., Philips E.J., Knight E., Drew M.K., Waddington G. (2018) Use of a tibial accelerometer to measure ground reaction force in running: A reliability and validity comparison with force plates. Journal of Science and Medicine in Sport.
-
Emily S. Matijevich, Lauren M. Branscombe, Leon R. Scott, Karl E. Zelik (2019) Ground reaction force metrics are not strongly correlated with tibial bone load when running across speeds and slopes: Implications for science, sport and wearable tech.